What is Mass Spectrometry and What are Isotopes?
- Maria Baias
- Dec 27, 2023
- 5 min read
Updated: May 16, 2024
Mass spectrometry is a very useful tool for analyzing isotopes and molecular fragments. Mass spectrometry has a large variety of applications in various fields, including cultural heritage. To learn what is mass spectrometry and how it can be helpful as a research tool, we first have to learn what is an isotope and how different chemical elements can have various isotopes.
What are isotopes?
One chemical element can have different forms that vary in their numbers of neutrons in the nucleus. These variations of a chemical element are called isotopes. To illustrate this, let's have a look at a simple example: lithium.
To understand isotopes, we have to understand two important numbers that define chemical elements based on their atomic structure. First, the atomic number, Z, gives us the number of protons in the nucleus. Second, we have the total number of protons and neutrons in the nucleus, which is given by the mass number, A.
Lithium isotopes

In the example above, we see two of lithium's isotopes. On the left, we have a lithium atom with an atomic number of 3 and a mass number of 6. This means that there are 3 protons in the nucleus, given by Z = 3. The number of neutrons in the nucleus is A - Z, so, for the lithium isotope on the left, that is 6-3, which is 3. This means it has 3 neutrons in the nucleus. But lithium can also have 4 neutrons in the nucleus (isotope on the right side), and this form of lithium still has Z = 3, so it still has 3 protons, but now A is 7 and that’s what gives us 4 neutrons in the nucleus.
These different forms of the same element that have the same number of protons, but different numbers of neutrons in the nucleus are called isotopes.
Lithium-6 and Lithium-7 are two of the lithium isotopes, both of them stable isotopes, lithium-7 being the most abundant lithium isotope. Besides these two stable isotopes, lithium also has many radioactive isotopes.
What is a radioactive isotope?
A radioactive isotope is an unstable form of an element because of its unstable nucleus. It regains stability through a radioactive decay, a process whose duration can be a few seconds or much faster than that for some isotopes, and can last as long as many billions of years and even longer for other isotopes. This decay time is called the half-life.
Let’s have a look at an example so we can understand this decay in time of radioisotopes.
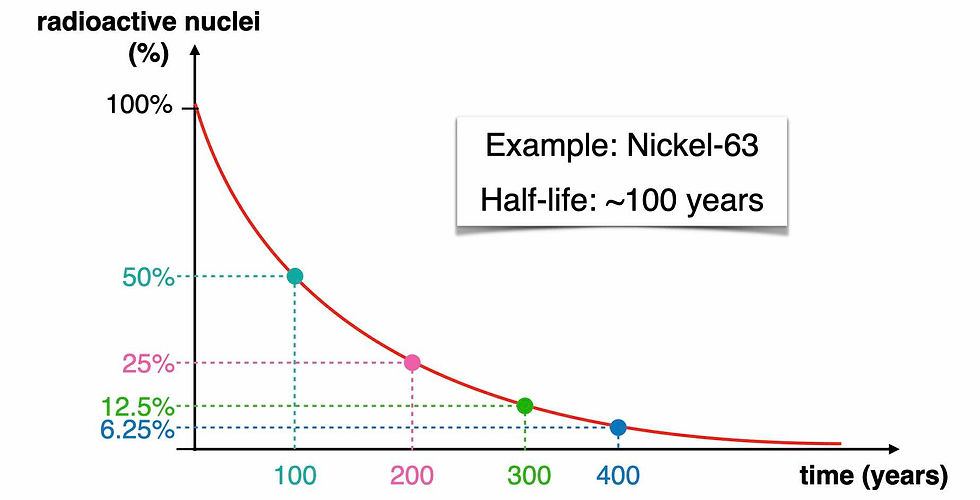
If we consider a radioisotope whose half-life is around 100 years, then after its half-life, so after 100 years, the number of radioactive nuclei we’re left with is half of what we had in the beginning. So after 100 years we’re left with 50% of the radioactive nuclei (the cyan dot in the graph). After another half-life, so after another 100 years, the new number of radioactive nuclei will be reduced again to half. That means that after 200 years, we’ll have only 25% of the initial number of radioactive isotopes (the pink dot). And every new half-life, in case of Nickel-63, every new 100 years, the number of radioactive isotopes is reduced again to half of what it was 100 years before.
Another example of a radioisotope is carbon-14 (radiocarbon), the carbon isotope that has 8 neutrons and 6 protons in the nucleus. This radioisotope of carbon is used in a scientific method called radiocarbon dating. This method is used to determine how old an object is, and it is very useful for detecting the age of objects made of organic matter, which is rich in carbon. Other radioisotopes can also be used for dating objects that contain that element.
What is mass spectrometry?
Mass spectrometry, or mass spectroscopy (Mass Spec) is the scientific method used to find out which isotopes and how much of each of them there is in a sample.
How does the Mass Spectrometer work?

First, we introduce the sample in the mass spectrometer.
Then we heat up the sample to vaporize it so that the sample would be in gas form. Then we ionize the sample, either by bombarding it with electrons or using chemical ionization or other methods to remove electrons from the atoms. This ionizes them and it gives them a charge.
Once the sample is ionized then the ions are accelerated and they move very fast until they enter a magnetic field. The purpose of passing these ionized sample beams through the magnetic field is to deflect the fragments based on their charge and mass. Fragments of the same charge but different masses will be deflected differently by the magnetic field. Molecular fragments, or isotopes with larger masses, will be deflected less, and those of smaller masses will be deflected more.
These differently deflected isotopes will hit the detector in different regions of the detector. By analyzing how much of the ions of each type reached different areas of the detector, we can find out how much of each is present. And that’s exactly what we can read in a mass spectrum.

This example illustrates the mass spectrum of zirconium. It has an atomic number of 40, so that means 40 protons in the nucleus, but it’s the number of neutrons that varies from one isotope to another. And by looking at the peaks in a mass spectrum, we can identify which isotopes are present and what is their relative abundance.
On the x-axis, we have the mass-to-charge ratio. When the charge is 1, meaning we have a +1 charge for the ions, then the x-axis represents the atomic mass. Here, we can see we have 5 different isotopes of zirconium, and we can see their masses on the x-axis. By looking at the peak intensity, we can find out the relative abundance of each isotope.
We can do the same kind of analysis when we deal with molecules.
In the mass spectrum of the acetone molecule, there are 4 different peak regions corresponding to different mass ranges. The peaks in these regions represent the different molecular fragments of different masses. The big peak at the highest mass represents the radical cation, that is simply the positive molecular ion of acetone.
The peaks in the other regions represent smaller molecular fragments that came from fragmenting the acetone molecules in different ways. The smaller fragments will be found at lower masses.
There are also some smaller, very low intensity peaks around the larger ones. Some of these could be coming from the fact that, not only do we have different molecular fragments with different masses, but we also have lower abundance isotopes of elements present. This can also lead to a small variation of the masses because of the different mass of different isotopes of the same element.
The analysis of a mass spectrum reveals the presence of different molecular weight fragments and how much of each we have in a sample. By combining mass spectrometry with other methods, we can identify the chemical composition of larger complex molecules.
Comments